Unfortunately, you don’t have access to this resource.
The RNS exists to bridge the gap between rheumatology healthcare professionals and patient education. We offer memberships to all healthcare providers in order equip each person with valuable local and national networking opportunities and resources for the benefit of the patient, family, and community.
As a member of the RNS, you can receive the benefits of our entire library of evidence-based education, booklets, online accredited activities, and more.
To get full access to all resources on the RNS website, please sign up for an RNS Membership and gain access today.
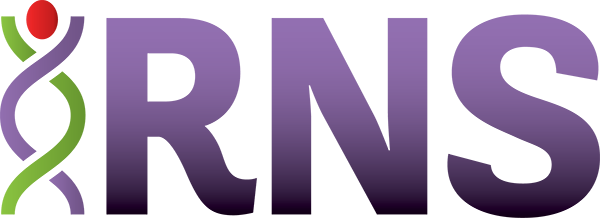